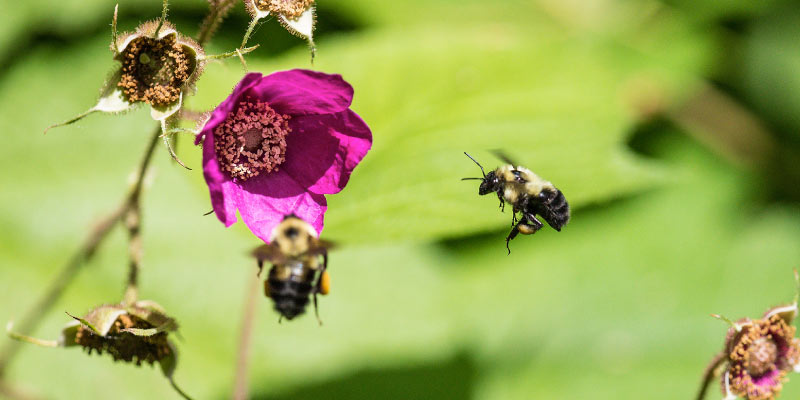
Have you ever wondered how insects fly? If you have, you’ve got good company in James Crall, researcher in Nelson’s Center for Ecology and the Environment and assistant professor in the Department of Entomology. (And if you haven’t, I bet you are now.) Crall started his academic career with a degree in sociology. “I basically fell into a biological research rabbit hole,” he says. “I was doing a project on these really fine details of insects and how they fly, which got me totally fascinated with how insects are put together and how they do what they do.” He took an intentional detour to work in a lab for a year, which turned into two years, which turned into a PhD in organismic and evolutionary biology from Harvard. His research started on the organismal scale: how insects fly in chaotic wind conditions. That translated into looking at whole bumblebee colonies, which piqued his sociologist brain: what are bumblebees’ social behaviors, and how are stressors like pesticides and climate change affecting them? “In some ways, getting interested in bees got me back to where I thought my original path was going to be,” Crall says.
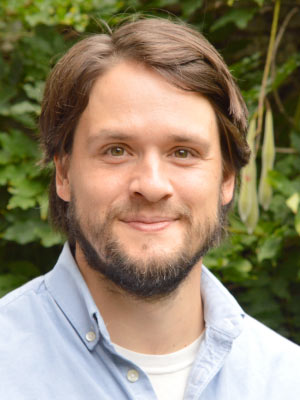
Wait … how do bees fly?
It’s cool! They have some very, very unique aerodynamics. The way in which they fly is very different than a plane and has to do with how small they are and the fact that they’re kind of rotating. It turns out that they’re very good at coping with these challenging natural environments. Their wings sort of flap back and forth really fast, like hundreds of times a second. It turns out they basically create little vortexes at the end of each wing strip. Their aerodynamics are very different than how a plan stays in the air. They’re kind of like these amazing little machines.
Why do you study bumblebees?
They’re probably our most important native pollinators in North America. They also have this cool annual social system. They build up a colony each year. You can think of them as an annual plant. They’re starting from a seed, essentially, and growing up each season again, but doing that colony growth every year. They’re an interesting system for thinking about how sociology might affect sensitivity. We often study them at that big, mature colony phase, but around here, in April or May, those colonies are just starting out. If we want to understand how the chemical or physical environment is going to impact bee populations, we’re largely focusing on the wrong stage.
So, colony size plays a part in their resilience?
We think that as colonies grow and get larger, they become more robust to environmental stressors. In particular, we’re looking at pesticides and temperature stress. As colonies are larger, it turns out that the ways in which they congregate and behave collectively, they’re able to buffer the combined stressors of climate and agrochemical exposure. That is, from a basic biology perspective, really fascinating.
How are pesticides affecting their social behavior?
Neonicotinoid pesticides are the most common insecticides used globally. They were introduced in the mid-nineties, and they’ve absolutely taken off. They target the insect’s central nervous system, disrupting a lot of really fundamental aspects of behavior. We have shifted significantly in the past couple decades to using these chemicals prophylactically; what we often do is incorporate the compound into seeds. The compounds are taken up systemically by the plant. If you’re a grower, that’s great, because your corn plant has resistance through the whole course of crop growth.
But why is that bad for pollinators?
Because the compounds are systemic, they show up in all parts of the plant, including nectar and pollen that bees feed on. Concentrations of these compounds will show up in nectar and pollen way below lethal levels of exposure, so the levels that are kind of considered safe by EPA standards. But those levels are mostly focused on toxicity. Work that we’ve done has shown that even really low levels of exposure disrupt all kinds of behaviors and processes, including social behaviors. Bees stop nursing, they stop interacting with each other, they shift out to the periphery of the nest, and they become less active. In the lab, we’re really interested in better understanding those impacts.
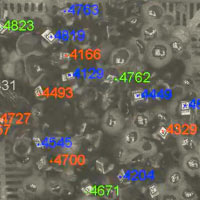
What are some of the local or global risks posed by those behavioral changes?
So there’s the answer to that within bumblebees, which is that we suspect it translates to impaired colony growth. It also might change how they respond to things like temperature stress. That’s one of the big concerns — that the compounds would change, within a season, what bees are doing and how good they are at delivering the pollination services we care about, but also long-term population health.
How is your lab investigating those effects?
How we quantify those effects is through computer-vision–based tracking of bees. We’ve set up systems where we can look inside of a colony and actually follow individual bees. We attach little QR codes to each bee and then can monitor their movements and what they’re doing, basically to quantify those low-level behavioral impacts.
Does the QR code’s weight affect their flight?
It’s very lightweight compared to what they can carry. They’re such good and efficient flyers. They will leave the hive with an empty tank, and then go pick up food and pollen, and the weight of that food and pollen substantially outweighs anything that we add. They’re the small tankers of the aerial insect world.
That’s so cool!
They’re amazing. The more you look at them, the more you’re like, “That ability to fly so far outpaces what we can do with any human technology.” They have all their weight and energy on board. They can maneuver through these wildly complex environments. They basically keep a map of which food resources are where in the environment and you’ll see the same bees come back to the same place every day because they have phenomenally good memories, but they also learn really quickly. They’re just amazing creatures. They learn from each other, they pay attention to who’s foraging on what. We often think of them, at first glance, as these simple automatons. But they are phenomenally complex and just endlessly fascinating, cool creatures.
That’s your work in the lab — what are you doing in the field?
One of the things we’re very interested in is taking advantage of low-cost electronics and rapidly improving computer-vision techniques. We’ve been building a low-cost, ‘smart’ pollinator camera: the idea is to have something you put out in the field, it watches a flower, and then it can actually identify when different bees are visiting, who they are, and maybe how they’re responding to different temperature conditions. We have a fun prototype we’ve built, and we have some of our first experiments going, which have been really exciting.
Are there any initial findings that are surprising?
There’s one surprising result that is a very early, tentative result. We did some work in apple orchards; this spring was our first test of this system. Apples are a cold-season crop, and they’re pollinated by largely solitary native pollinators: some bumblebee queens, sometimes with honeybee supplementation. We happened to be doing those experiments during a substantial heat wave this spring. We often think of insects as being very heat tolerant, but it looks like during that heat wave, there were substantial reductions in visitation, even in that early part of the season. This is something we’re becoming very interested in: with heat waves and increased temperature fluctuations here in Wisconsin, could we be experiencing even less time in the sweet spot for a lot of our crops where there’s a lot of good pollinators around?